Mitigating and adapting to climate change are key challenges of the 21st century. At the core of these challenges is the question of energy — more precisely, our overall energy consumption and our dependence on fossil fuels. The best course of action to mitigate the impacts of climate change is a transition away from fossil fuels, particularly in the transport and energy industries.
These two sectors are currently in the early stages of transition, but they are responsible for the vast majority of carbon emissions driving climate change.
Electric vehicles (EVs) are set to take over the automotive market in the coming decades, and an increasing amount of utility companies are adopting renewable power sources. The key to these transitions resides not only in the energy source but also in how that energy can be stored.
Electric Vehicle Battery Technologies Driving Change
The transition to carbon neutrality and renewability is well underway—and batteries have an important role to play. While renewable energy sources such as solar and wind power are increasingly replacing fossil fuels, batteries have emerged as a viable storage option for power grids of the future.
However, the development of battery technology is being driven by the shift toward electric transportation is also driving the development of battery technology. The rise of batteries as a tool to transition towards cleaner energy and sustainable development will reduce the amount of greenhouse gases released by using fossil fuels.
The United Nations Development Programme (UNDP) and the Intergovernmental Panel on Climate Change (IPCC) have identified the lack of power storage options as a major brake on the transition towards renewable energy to mitigate climate change. All that is set to change.
The Growth of the Energy Storage Industry
Energy generation from renewable sources like solar, wind, and hydro, does not always coincide with the energy demand. Therefore, more advanced methods of storing energy have become critical in recent years (Divya and Østergaard, 2009).
Batteries are integral to our modern world. They serve as essential components in innumerable devices (Ziglar, 2019). Modern lithium-ion batteries have revolutionized our day-to-day lives, powering everything from smart mobile devices to pollution-free electric vehicles. Over time, they have become increasingly efficient, and new battery technologies offer enormous potential for increasing the spread of electric vehicles and storage for large scale energy grids.
Despite these successes, gaps in battery technology remain, both in terms of safety as well as performance. These core issues are driving not only the research into new battery materials but also improvements in efficiency.
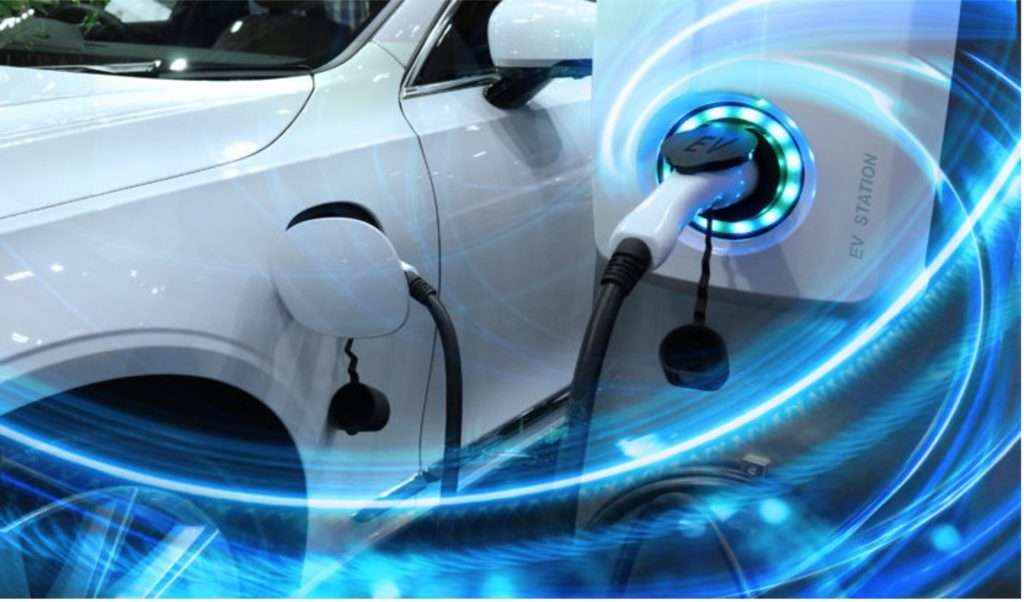
The Lithium-ion Battery
Significant advances have been made in battery performance, but they still have not reached a level that makes many innovations viable.
Lithium-ion batteries are the most widely used battery storage device. They have high specific energy, single-cell voltage, energy efficiency, and long life spans, and are excellent sources of power (Horiba 2019).
Lithium-ion became the go-to form of energy storage because they have an extremely high electron density, which means it can store a lot of energy within a relatively small volume.
The Hornsdale Power Reserve in South Australia has provided the blueprint for the implementation of large-scale batteries into major power grids. Built by Tesla as part of a challenge issued by Elon Musk to the Australian state government, lithium-ion batteries have entirely revolutionized the energy storage industry. Within a year of operating, the reserve saved the state from several blackouts, generated a profit from the sale of stored energy during peak power demand, and reduced costs for utility companies and consumers alike.
Lithium in Electric Vehicle Battery Technologies
Lithium itself is the lightest metal on the periodic table, which makes lithium-ion batteries extra portable. However, since the material has been incorporated into electric vehicle battery technologies, these batteries have been pushed to their limits.
Lithium-ion batteries can only be charged and discharged so many times, and we may have hit the upper limit of their storage capacity. This is one of the biggest concerns that people have with EVs since more capacity equals a longer driving range. In addition, batteries currently take up a huge amount of space in electric cars, which limits how much additional range can be added.
Therefore, electric vehicles with this kind of battery technology are still unable to match the performance of a vehicle driven by internal combustion engines (Coffelt et. al., 2019).
How do Electric Vehicle Batteries work?
EVs aren’t powered by one big battery, but rather by thousands of smaller cells. Each cell has four key components: an anode, a cathode, a separator, and an electrolyte, which is usually a liquid. To power a device like a car, charged atoms or molecules called ions move from the anode to the cathode through the electrolyte, releasing their extra electrons along the way and thereby producing electricity.
When charging a battery, the opposite happens: Electrons flow into the battery, and the ions flow back from the cathode to the anode, creating potential energy that the battery must store for later use. In the case of lithium-ion batteries, these ions are, naturally, lithium ions.
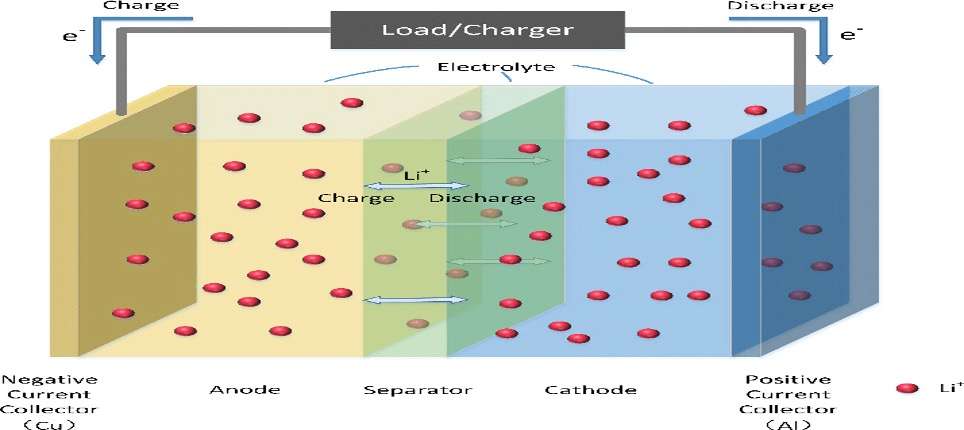
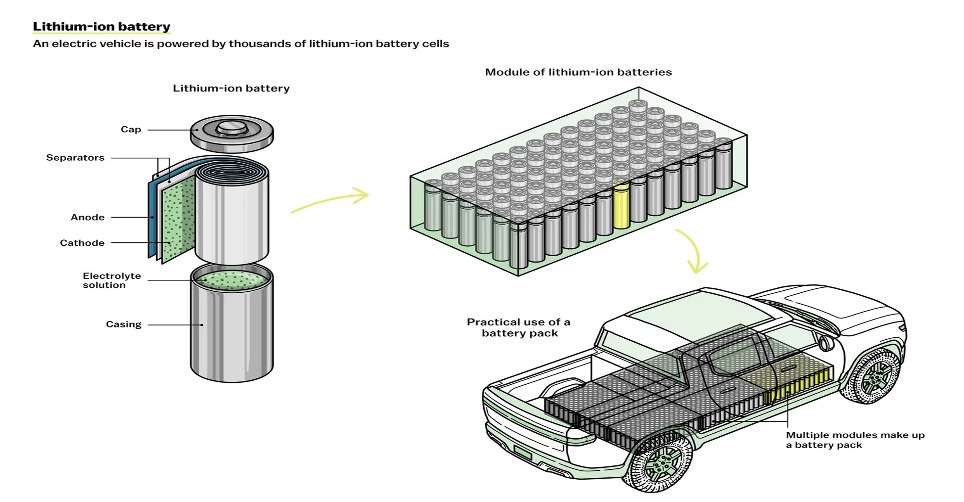
The first lithium-ion battery was used to power one of Sony’s camcorders, and this battery technology quickly became ubiquitous in consumer electronics. Automakers turned to lithium-ion batteries to power their electric cars largely because they were so widely available.
Dozens of lithium-ion battery cells are packed into larger protective shells, called modules. These modules are then assembled into an even larger battery pack, which provides the power to the EV.
Problems with Electric Vehicle Battery Technologies
If the EV revolution is going to succeed, electric vehicle battery technology needs to get better. Firstly, they need to be able to go even farther on a single charge, and they need to weigh much less. Also, EV batteries need to be less prone to bursting into flames. It’s an issue that’s still quite rare, but nonetheless very concerning.
Car maker, Chevy, recently had to recall every Chevy Bolt it had ever sold due to a battery fire risk.
According to Dragonfly Energy CEO, Dr Denis Phares, solving the issue of flammability is the greatest challenge facing electric vehicle battery technologies. The current Lithium-ion battery falls well short of the mark.
The other major limitation is range. The average electric vehicle has a range of 260 miles. That’s enough to get around for day-to-day trips, but makes a lot of drivers worried about longer-range excursions.
The Availability of Materials for EV Batteries
Lithium itself has some problems, too. Lithium mining isn’t particularly environmentally friendly, and right now the world doesn’t have enough lithium mines to supply enough material for the number of EV batteries we probably need.
Also, there are mounting concerns with other metals commonly used in the production of lithium-ion batteries; namely cobalt. Cobalt is mainly extracted in the Democratic Republic of Congo and has been linked to child labor and human rights concerns.
The scarcity of resources, and related problems in the supply of these batteries, present major issues that must be solved or almost completely minimized before their continued use can be seen as sustainable (Coffelt et. al., 2019).
For example, the toxicity of the processes used in extracting the raw materials is harmful to both the environment and human health. Also, the rarity of many of the materials used violates the principles of responsible production. Depletion of resources and the degradation of land lead to economic instability.
Currently, lithium-ion batteries cannot continue as our primary means of portable energy storage.
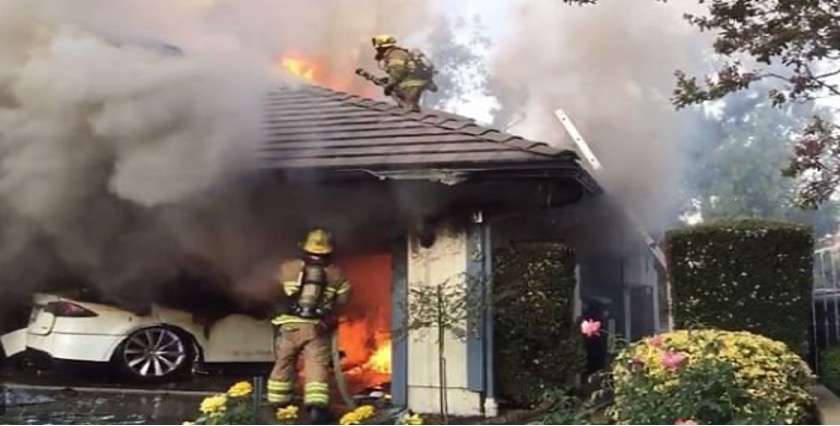
Graphene in New Electric Vehicle Battery Technology
Therefore, whilst our current batteries are not long-term solutions, alternatives are being explored. New and novel materials are being tried that could be used in composites to increase the efficiency of specific components, or to construct completely new battery designs.
One such material is graphene, which was discovered only recently, even though its use had been theorized for many years (Coffelt et. al., 2019). Graphene has remarkable qualities due to its unique structure and properties.
Although still not fully understood, graphene is being applied in electronic and energy systems, where the material has demonstrated greatly improved efficiency and safety. Importantly, graphene seems to address some of the practical limitations of lithium-ion batteries, like charging speed and capacity.
Also, while functionality is important, the key role that batteries play in our society means that sustainable extraction and production methods are imperative (Coffelt et. al., 2019).
Graphene as an alternative component in batteries
Since it was first isolated as a material in 2004, graphene has held great interest (Randviir et al., 2019).
Currently, graphene is the most studied material for charge storage. The results from many laboratories confirm its potential for transforming today’s energy-storage landscape.
In particular, graphene might offer new features for energy-storage devices, including smaller capacitors, completely flexible, and even rollable qualities, transparency, high capacity and significantly faster charging (El-Kady et al., 2016).
In fact, graphene’s unique properties offer great potential for a variety of applications across myriad sectors. Researchers have been attempting to incorporate graphene into numerous electrical and energy systems, with batteries being one of the most well-known.
What is Graphene Made Of?
Graphene is an allotrope, or one of the various physical forms, of carbon. This makes it similar in structure to materials like diamond, coal, and graphite.
Specifically, it is a two-dimensional sheet of carbon atoms, arranged in a honeycomb-lattice or hexagonal structure (Randviir et al., 2019). This characteristic structure arises from the sp2 hybridization of its carbon atoms, which creates a trigonal planar geometry where each carbon atom is bonded to three others (Neto, 2019) as pictured below:
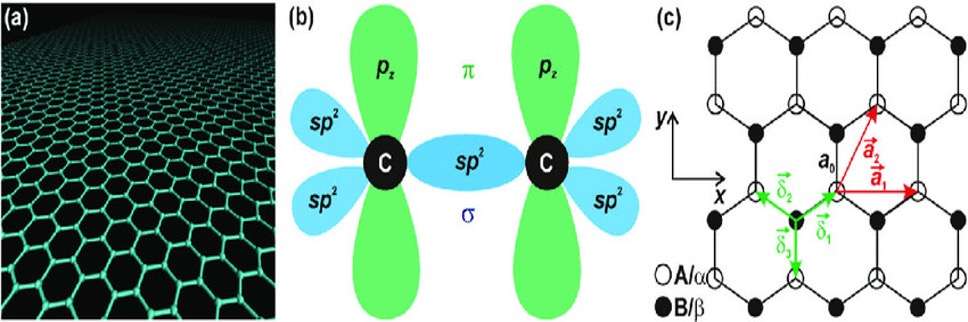
(c) The honeycomb lattice structure of graphene.
Source: Jiri Tucek et al., 2017
What Makes Graphene so Useful for Electric Vehicle Battery Technologies?
Graphene’s two-dimensional structure offers a number of qualities, beyond sheer strength, that make it appealing to energy research. One characteristic is its large theoretical specific surface area (SSA) of approximately 2630 square meters per gram (m2/g) (Zhu et al., 2019). This quantity, the ratio of surface area per gram of substance, is particularly important in battery components. It allows them to store and release charge carriers—vital to any component dealing with energy storage (Bonacorrso et al., 2019).
The more charge carriers (like Li-ions) that a material can store and release, the greater the storage and rate of energy transfer (M. Cao et al., 2019).
Additionally, graphene exhibits unparalleled electrical conductivity, which is essential for the next generation of electric vehicle battery technologies (S.K. Tiwari et al. 2020). Further, graphene has excellent optical, thermal, and mechanical characteristics (Flynn 2015). 2.3% of white light is absorbed by each layer of graphene with a reflectance of less than 0.1%. Thus, a pure, single graphene layer is highly transparent and offers a high degree of flexibility.
Even at this lower conductivity, graphene performs much better as compared to copper (Cu). Thus, graphene is the strongest and best conductive material (Flynn 2015).
Samsung’s ‘graphene ball’ battery
Recently, a team of researchers at the Samsung Advanced Institute of Technology (SAIT) developed a ‘graphene ball’, with a 45% increase in capacity, and five times faster-charging speeds than standard lithium-ion batteries.
According to Samsung Newsroom, the battery “provides promise for the next generation secondary battery market, particularly related to mobile devices and electric vehicles”. Considering Samsung’s recent history, this advancement would be particularly relevant to the smartphone industry. Also, the battery is able to maintain a stable temperature of 60 degrees Celsius, which makes it ideal for use in electric vehicles, possibly in future models of Tesla’s electric cars.
For this development, the Samsung Advanced Institute of Technology (SAIT) collaborated closely with Samsung SDI, as well as a team from Seoul National University’s School of Chemical and Biological Engineering. In theory, a battery based on the ‘graphene ball’ requires only 12 minutes to fully charge. Additionally, the battery can maintain a highly stable 60-degree Celsius temperature (Girish et al. 2019).
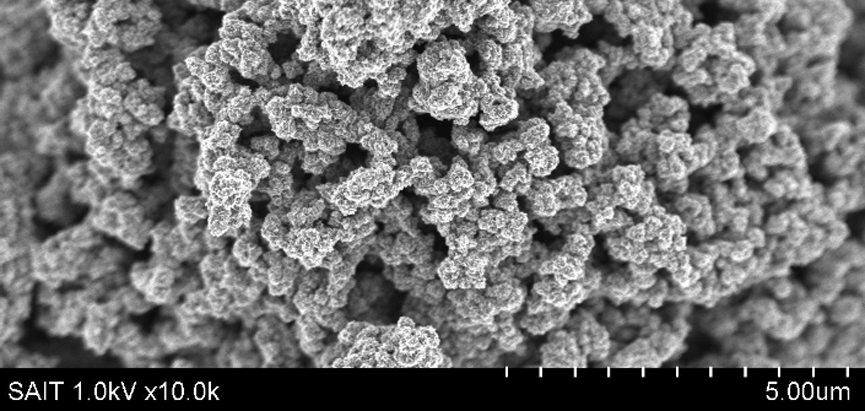
Source: SAIT
Mass Production
By synthesizing large quantities of the material, Samsung will be able to maintain relatively low prices for both graphene and the production of batteries. This is a great step for the future of graphene, which may encourage other companies to follow suit (Jared Coffelt et al., 2019).
It seems likely, therefore, that the smartphone, electric vehicle, and broader electronic industries will soon switch to a battery similar to Samsung’s prototype. Although not yet commercially available, Samsung’s battery demonstrates that graphene-based batteries do have clear commercial applications.
The graphene ball is not only more effective than other graphene batteries in terms of capacity and charge time, but it is also less expensive and more suited to mass production.
As a leading technology company, Samsung’s use of the battery could serve as the catalyst for the broad adoption of graphene in many energy storage applications.
Other Potential Applications for Graphene
Because of its high conductivity and open porosity, graphene also offers potential for supercapacitors. The material is electrochemically stable and has a higher surface area than activated carbon, the material used in supercapacitors today (Sur, 2012).
Because graphene is extremely thin and lightweight, it can be made into a paper-like material and used to create flexible or rollable batteries.
In addition, graphene is well suited to the manufacture of solar panels because of its high conductivity (Sur, 2012). Further, graphene and graphene-based hybrid nanostructures are attracting interest as novel materials for nanotechnology, biomedical engineering, material science, physics, and green chemistry. This is due to their tunable physical properties, high surface area, elevated electronic, and thermal properties. Thus, within a very short period of time, graphene and its derivatives have shown wonderful commercial applications in the field of composites, nanoelectronics, bioimaging, and nanomedicines (S.K. Tiwari et al. 2020).
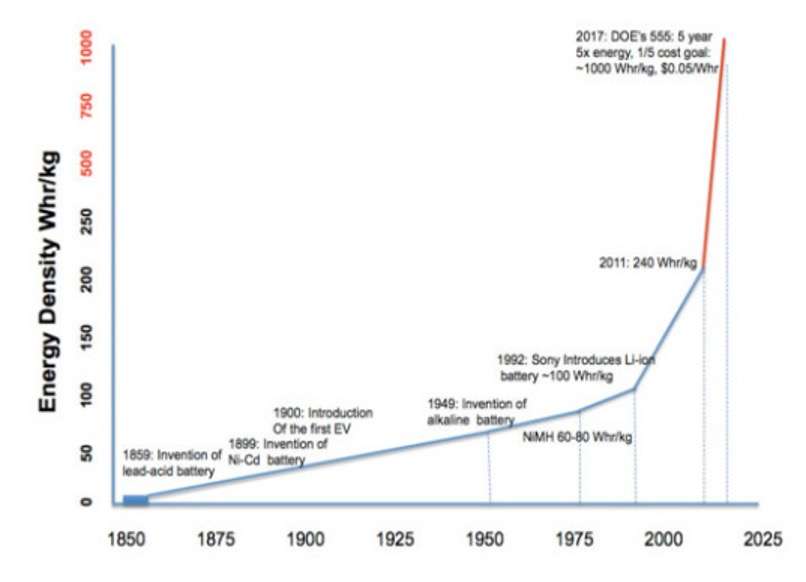
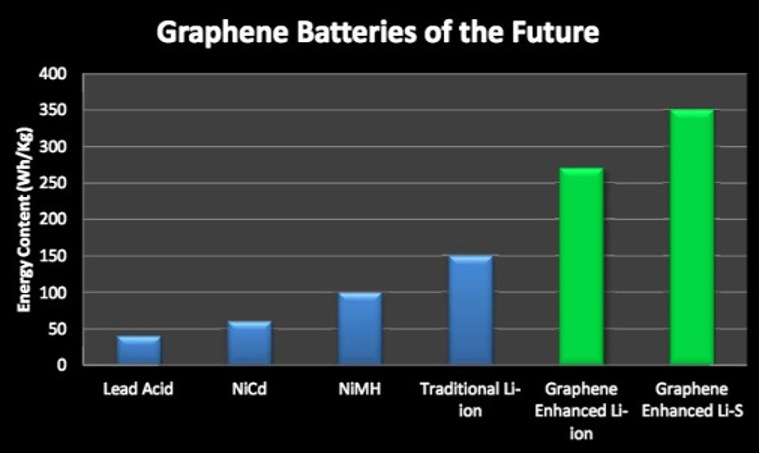
The Limitations of graphene and graphene-enhanced batteries
Despite its promising characteristics, and its potential to solve issues of sustainability, (Coffelt et. al., 2019) the greatest limitation of graphene-based battery technology is the lack of available mass production.
The cost ranges from tens to thousands of dollars per kilogram. That amount is significantly higher than the cost of producing activated carbon at $15 per kilogram (Lamuel et al., 2016).
Moreover, the thickness of graphene-based materials is generally limited to micrometers. This limits the overall battery capacity significantly. Lastly, graphene batteries show very high first cycle loss at 50%-60%, low cycling efficiencies at 95%-98%, and poor capacity retention at high current densities (Lamuel et al., 2016).
Moving Forward
While many believe graphene has great possibilities, further research is needed to determine the practical capabilities of this material. Mass production remains a high priority if graphene is ever to be considered for commercial applications. In the near term, composite materials are probably the most practical application for graphene (Sur, 2012). It will likely become a crucial material in future electronics and large-scale energy storage.
Taking everything into account, transforming vehicles to battery power remains a herculean task. However, it isn’t the only one. In order to transition away from fossil fuels, we need to switch to renewable energy sources, like solar and wind power. Yet, when the sun and the wind aren’t available, we need methods of storage that are safe, fast and effective.
That means our homes, cities, and even the electric grid will need high-capacity batteries. These won’t necessarily have the same needs as the batteries used in cars, or those that power our phones. However, they’ll all play a critical role in the transition away from fossil fuels and mitigation of climate change.
The Thrive Framework
At THRIVE we believe that there is no truly sustainable energy system in an unsustainable world. The Thrive Framework has been developed to assess the impact of alternative strategies toward sustainability.
At its core, sustainability simply means the ability to continue, to survive. ‘Thrivability‘, by contrast, is the next step, beyond sustainability. THRIVE believes that humanity can do better with the knowledge currently available to us. We want to instil the idea that sustainable solutions will not only prevent climate disaster but offer the potential for societies to flourish.
The THRIVE Framework examines issues and evaluates potential solutions in relation to this overarching goal of thrivability. It is about making predictive analyses using modern technology that support environmental and social sustainability transformations.
To learn more about how The THRIVE Project is researching, educating and advocating for a future beyond sustainability, visit our website. However, you can follow our informative blog and podcast series, as well as find out about our regular live webinars featuring expert guests in the field. Sign up to our newsletter for regular updates.
GOT A QUESTION ON THIS TOPIC?
Talk to an Expert
OFFLINE REFERENCES
K.C. Divya and J. Østergaard (2009), “Battery Energy Storage Technology For Power Systems – An Overview,” Electr. Pow. Syst. Res. 70, 511.
Maher F. El-Kady, Yuanlong Shao and Richard B. Kaner (2016); “Graphene for batteries, supercapacitors and beyond.” Article number: 16033, doi:10.1038/natrevmats.2016.33.
G.W. Flynn (2015), Atomic Scale Imaging of the Electronic Structure and Chemistry of Graphene and Its Precursors on Metal Surfaces, Columbia Univ., New York, NY (United States).
Girish K, Karthik Raj H P, Manoj M (2019); “Graphene Batteries over Lithium Ion Batteries.”, National Conference on Communication and Image Processing 2019, ISSN (Online): 2348-4098, International Journal of Science, Engineering and Technology.
T. Horiba 2019; “Lithium-Ion Battery Systems.” Proceedings of the IEEE. Vol. 102, pp. 939–950, 2014.