THRIVE Framework
Finite Resources
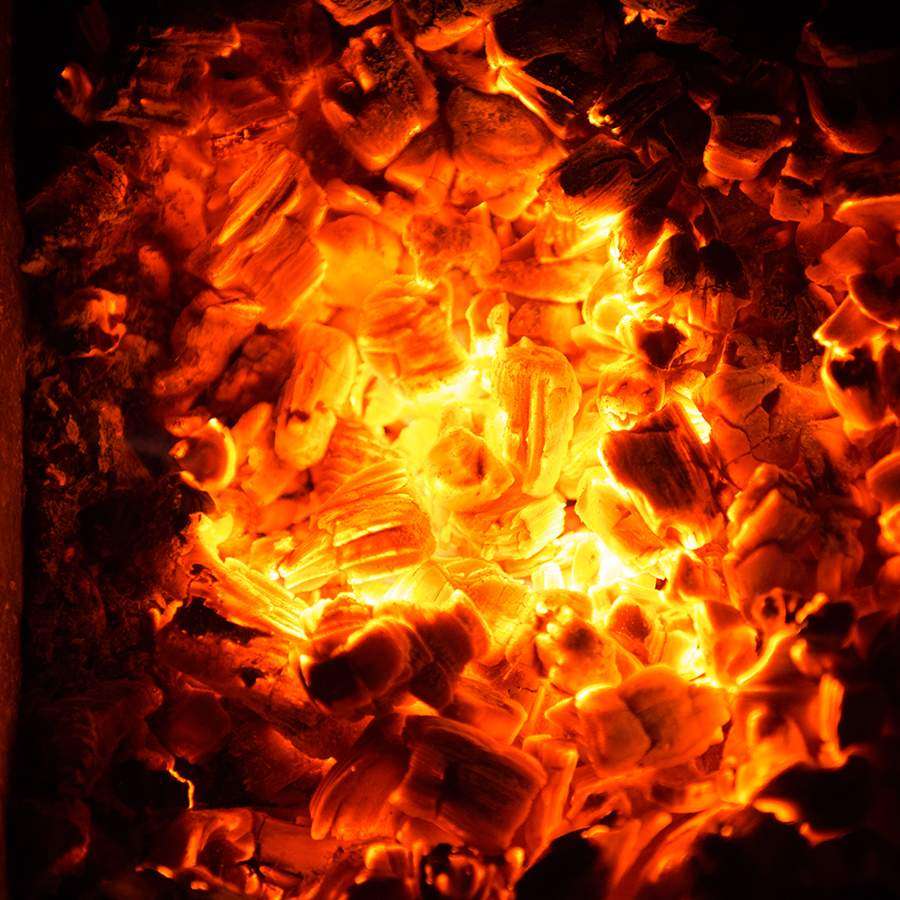
Burning coal. Coal is a finite resource.
Source: Valuavitaly on FreepikCo
Finite resources: A definition
Natural resources are elements or substances that are found in nature and can be used for economic advantage. Examples include minerals, forests, fresh water, and fertile land. They may or may not be finite or limited. Thus, in simple terms, finite resources are non-renewable natural resources present on Earth. Resources that we consume faster than they can be replaced are called non-renewables. As non-renewable resources are finite resources in nature, the sole option is to minimise depletion through reduction, reuse, and recycling (also known as the three Rs).
One such non-renewable is fresh, drinkable water. Drinkable water is only 1.2% of the total water available on earth for the entire world population of 8 billion people. Similarly, cobalt is a rare earth element used in lithium-ion batteries. These batteries are used in electric vehicles, phones, tablets, phones, etc. Cobalt mining has widely been linked to child labour exploitation, exposure to hazardous substances, greenhouse gas emissions, and environmental pollution. Coal and oil are some of the major contributors to greenhouse gas emissions. These are also finite resources that take thousands of years to form from dead plants and organisms.
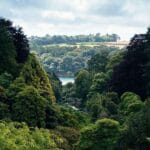
A vertical shot of thick forest and a river.
Source: Freepik.
Non-renewables as a foundational focus factor: finite resources
Against this backdrop, how can real progress be made to reduce depleting finite resources? How can we measure the rate of resource depletion without knowing the impact on non-renewables? A systemic holistic framework and platform like the THRIVE Sustainable Performance Scorecard (SPS) make it viable. The THRIVE Framework is a trans-disciplinary, holistic model, used to analyse sustainability-related issues, Sustainable Development Goals, and explore sustainable solutions.
THRIVE Project aspires to evince a “thrivable” zone using a Ciambella Chart and a systemic holistic model, which includes 12 Foundational Focus Factors. The Ciambella Chart outlines the minimum, known as the social floor, and the maximum, known as the environmental ceiling. The social floor reveals the minimal requirement for communities. The environmental ceiling marks the upper limit of environmental harm allowed before it becomes unsustainable.
One of THRIVE’s 12 Foundational Focus Factors (FFF) is the topic of Finite Resources. This is based on the fact that finite resources are non-renewable. It also elaborates on why it’s essential for us to measure the usage relative to how much exists. Rockström et al., (2009) identified the nine planetary boundaries as climate change; ocean acidification; stratospheric ozone depletion; atmospheric aerosol loading; biogeochemical flows (nitrogen and phosphorus cycles); global freshwater use; land system change; rate of biodiversity loss; and chemical pollution.
What is the significance of Finite Resources?
The significance of non-renewables is critical as the escalation in human action has already substantially impacted Earth’s ecosystems (Crutzen, 2002). Thus, the current geological time interval in human history where humans are the dominant driver of the ecosystems is unofficially referred to as the Anthropocene Epoch. Humankind has already crossed the boundaries for climate change, rapid extinction of biodiversity, and high interference of the nitrogen cycle through human activities such as the usage of nitrogen fertilisers (Raworth, 2012). Once a boundary is transgressed by humans, the feedback period is uncertain (Rockström et al., 2009). Yet, the shrinking Arctic sea ice is evidence of the fast feedback of contravening the climate limit.
The Anthropocene Epoch was invented by biologist Eugene Stormer and chemist Paul Crutzen in 2000. He used this term to describe the latest era in human history. It still hasn’t been formally approved by the International Union of Geological Sciences (IUGS). The IUGS classifies these units based on stratigraphy. However, the Anthropocene Working Group (AWG) accepted that the Anthropocene is different to the current formal geological epoch of the Holocene. They also identified that the Anthropocene Epoch began in the 1950s. This has stemmed from the rapid human-driven trends called the ‘Great Acceleration’ of population growth, socioeconomic expansion, and globalisation (Shoshitaishvili, 2021).
Continued pressure on Finite Resources
The continued boom in human deeds is further adding pressure on the ecosystems of the Earth (Steffen et al., 2015). This can disrupt the biophysical environments and initiate sudden and lasting changes to the ecosystems that would be deadly or disastrous to humanity (Rockström et al., 2009). In 2022, Earth Overshoot Day fell on July 28. This means it took less than eight months for humanity to exhaust all the natural resources and non-renewables Earth can regenerate within a year. For the remaining year, humanity will accumulate ecological debt. This will be by further depleting the natural resources and raising the atmosphere’s carbon dioxide content.
How did humanity begin to put a strain upon these Finite Resources?
Climate Change and Impact on Finite Resources
Human activities have resulted in global warming and increased the greenhouse effect. These activities include burning fossil fuels, deforestation, and farming livestock:
- Burning coal, oil and gas to generate energy produces greenhouse gases.
- Livestock, such as cows and sheep, release substantial levels of methane during food digestion.
- Fertilisers comprising nitrogen emit nitrous oxide.
- Gadgets emanate fluorinated gases.
- Destroying forests obstructs the natural effect of trees absorbing the CO2 from the atmosphere. It compounds the problem by releasing the CO2 stored in the trees into the atmosphere.
Thus, human-induced climate change is dangerous to humankind. Human-induced CO2 is the main contributor to global warming. By 2020, its accumulation in the atmosphere had climbed to 48% above its pre-industrial level. The global average temperature rise of 2°C, in comparison to the pre-industrial era, can have detrimental effects on the ecosystem, human health, and wellness. This escalates the potential threat of catastrophic climate events in the environment.
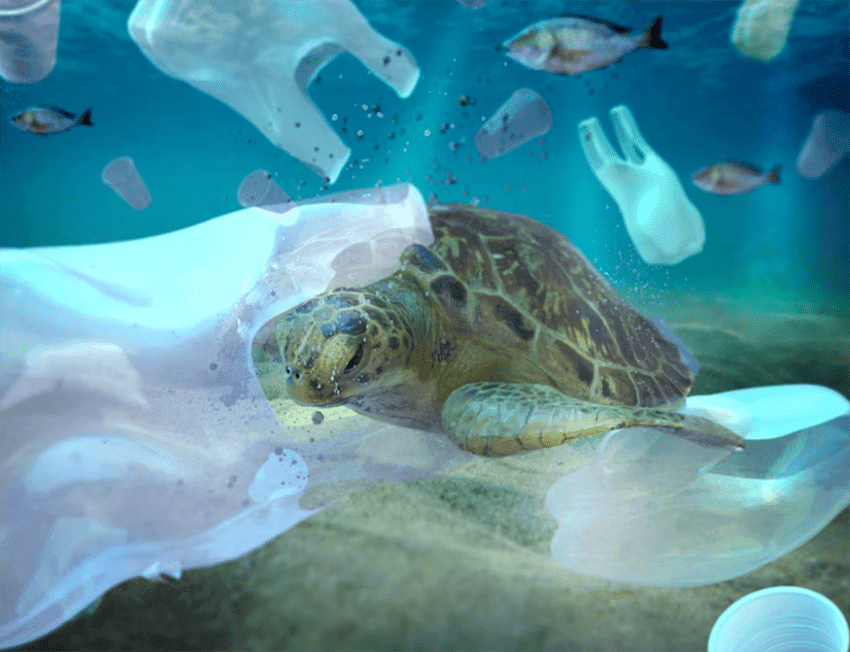
Image of turtle swimming amongst plastic pollution.
Source: Freepik.
Ocean Acidification and Impact on Finite Resources
Ocean acidification refers to the decline in the pH of the ocean over a long period. This is due to the fact that oceans absorb about 30% of the carbon dioxide released into the atmosphere. The amount of CO2 in the ocean has risen in correlation with the increased carbon dioxide content in the atmosphere. Human activities have driven the amount of carbon dioxide released into the atmosphere. These activities include burning fossil fuels and changes in land use. Oceans have become 25% more acidic since the industrial revolution. This is shifting the ocean’s chemistry and marine life, which could have adverse impacts.
Seawater becomes more acidic when it is saturated with carbon dioxide from the atmosphere. Furthermore, rising temperatures disrupt carbonate ions, which are essential for the formation of sea shells and coral. This further hinders the process of calcification for marine organisms. These organisms include oysters, clams, sea urchins, shallow-water corals, deep-sea corals, and calcareous plankton.
Calcification is the mechanism by which these organisms combine calcium and carbonate in the ocean. Organisms do this to build hard shells and skeletons, from calcium carbonate, for structure and defence. For example, the corals serve as a habitat and food for a variety of marine species. Acidic oceans impede the growth of these calcium carbonate structures. This threatens the entire ecosystem. In extreme cases, these could even dissolve quicker than they form.
Impact on Food Web
The elevated acidic conditions in the oceans also impair the health of marine species. These marine species need to use more energy to counter the adverse effects of this acidity. For instance, mussels, sea urchins, and crabs begin to dissolve their defensive shells. They do this to balance the raised acid levels in their body fluids. Some marine species lose their ability to identify their predators in acidic oceans. This can diminish the stock of these life forms and impact the entire food web.
Meanwhile, some organisms may rise in abundance. Seagrass is one such example that may grow rapidly due to the increase in dissolved carbon dioxide. This imbalance will ultimately affect the food chain. Every organism in the ocean is always food for another. Hence, ocean acidification can change the ocean chemistry. This, in turn, can have a domino effect on other plants and animals in the ocean for generations. Eventually, it will hit humanity, as humans depend on the oceans for a variety of significant factors. These include food, coastal protection, and economic activities like tourism.
Stratospheric Ozone Depletion and Impact on Finite Resources
The gradual thinning of the ozone layer in the upper atmosphere is referred to as ozone depletion. Ozone depletion is caused by the chemical substances comprising gaseous chlorine or bromine. Industrial work and other human actions release these substances into the atmosphere. The manufacturing of some products uses these ozone-depleting substances, such as chlorofluorocarbons (CFCs) and hydrochlorofluorocarbons (HCFCs). These products include refrigerators, air conditioners, fire extinguishers, foam, aerosol propellants, and fumigants.
The polar regions, especially over Antarctica, are where the ozone depletion is severe. Ozone depletion is a serious environmental threat that can affect human health. This proliferates the amount of ultraviolet (UV) radiation that enters the Earth’s surface. Exposure to this radiation is harmful as it escalates certain types of skin cancers, eye cataracts, and immune deficiency disorders. UV radiation also distresses the planet’s ecosystems by affecting plant growth and food webs in the marine and natural cycles.
The concerted global action to reduce the production and consumption of about 100 man-made ozone-depleting elements has been fruitful. Since countries pledged to take action under the Montreal Protocol adopted in 1987, they have reduced about 98% of these substances. Consequently, the ozone layer has shown preliminary signs of recovery. It will, however, be an extremely slow process that warrants diligent action aiding continued recovery. Humans must do further research in order to phase out the ozone-depleting substances that are still in use. For example, methyl bromide is still used as a quarantine fumigant. Also, halon is still used to extinguish fires in limited areas like aeroplanes.
Atmospheric Aerosol Loading and Impact on Finite Resources
When fine particles or liquid droplets are suspended in a gas, these are called aerosols. Aerosols can be naturally occurring, like dust, smoke, plant pollen, and so on. Otherwise, it is possible for humans to induce them. These kinds of aerosols are known as anthropogenic aerosols. Such activities include burning fossil fuels, wood, and charcoal, farming the land, manufacturing goods, extracting metals from rocks, and using household cleaning products. Aerosols have a cooling effect on Earth through trapping heat and forming clouds. They also pollute the air quality and can cause adverse effects on human health. These include dust, soot from fires, and chemicals emitted by industries.
The complexity of atmospheric aerosol loading and its impact on Earth and climate change is still the least understood and hardest to model. Yet, it is important to model and understand this interaction to precisely use the aerosols beneficially and to guard against any possible disastrous effects on humanity.
Biogeochemical Flows (Nitrogen and Phosphorus cycles) and Impact on Non-Renewables
Biogeochemical cycles are the channels between which chemical elements and composites circulate amid the life forms and the natural environment. These chemical elements include carbon, phosphorus, nitrogen, and sulphur, while composites consist of compounds like water. These cycles can shift when humanity manufactures or consumes these elements or compounds in differing amounts.
Nitrogen Cycle
The nitrogen cycle is the pathway through which nitrogen flows between life forms and non-living parts of the planet. It’s important to note that nitrogen is critical for plant growth. Though there is ample nitrogen in the atmosphere and soil, it is not in a usable form for plants to absorb. Bacteria help the plants to use nitrogen by making it available to them. Yet, this naturally occurring component is insufficient to harvest and feed the growing population. Therefore, nitrogen fertilisers make it available in usable form in large quantities to boost agriculture production. This is beneficial to grow crops even in land areas that were previously unproductive. However, overuse of these nitrogen fertilisers is detrimental to the environment.
The nitrogen that sinks in the soil ends up in the water bodies and causes eutrophication. Eutrophication means that the uncontrolled growth of weed plants and algae clogs the rivers, lakes, seas and oceans due to enriched minerals and nutrients. When a large quantity of unwanted algae grows and decomposes, it uses up substantial amounts of oxygen in the water during the process. Therefore, sea animals that cannot move to other locations in the ocean end up dying due to a lack of oxygen which is essential for their survival. This creates dead zones.
Ocean Acidity and Impact on Marine Life
The decomposition of the algae and plant matter also releases carbon dioxide into the ocean, reducing its pH levels. This contributes to ocean acidification. Acidic oceans impede the growth of aquatic creatures, like fish, and the formation of shells for species like shellfish. This reduces the stock of fish and other marine species, leading to biodiversity loss. It also poses food insecurity to those who depend on seafood.
The Earth’s atmosphere is mostly made of nitrogen. Other gases contain nitrogen in small quantities. These gases, such as Nitric oxide (NO) and nitrogen dioxide (NO2), contain molecules that are released during the burning process of car engines, power plants, and factories. In turn, when burned, these molecules impact the planet. When these molecules combine with other elements or compounds, they can contribute to smog, acid rain, and more. The freed oxygen atoms from nitrogen oxide, due to sunlight, can combine with oxygen molecules to form ground-level ozone, which is disastrous.
Phosphorus Cycle
Phosphorus is an essential element in transferring energy between life forms, the structure of the genetic material, and cell membranes, bones, and teeth. The available phosphorus often restricts the ecosystem efficiency and biomass when other resources like light and water are in abundance. Hence, humans mine phosphorus primarily for fertilisers to improve soil quality for cultivation. Phosphorus is mainly available in the earth’s crust. It erodes due to weather conditions like wind and precipitation. Then it enters the soil and ocean, changing the ecosystem. Bank erosion during floods can also transport phosphorus to rivers and oceans.
Similar to nitrogen, phosphorus in fertilisers also leads to eutrophication. Apart from that, plants absorb phosphorus from the soil and transfer it to animals who eat it for their survival and growth. Through animal excretions and dead organisms’ decomposition, phosphorus enters the soil and water bodies. Hence, apart from mined phosphorus in fertilisers, phosphorus also ends up in the ecosystems through detergents and sewage waste. The increased amount of phosphorus in the groundwater is also a concern as it often discharges into surface water, affecting water quality.
Global Freshwater Use and Impact on Finite Resources
A limited resource with rising demand is water. Fresh water is necessary not only for drinking but for sanitation, cooking, growing food, energy, and transport. Fresh water is also important to provide natural flood protection, trash disposal, and erosion prevention. Although 70% of our globe is covered by water, only 2.5% is drinkable by animals and humans. 99% of this freshwater is trapped in glaciers. This means 0.007% of global water is available to 8 billion people.
The concept of water scarcity is relative. Water availability fluctuates according to supply and demand, which changes throughout time. The infrastructure and water resources of many nations are unable to keep up with the demand as the world’s population rises and resource-intensive economic development continues. As demand rises and/or the quantity or quality of the water supply declines, there is an increase in water scarcity. The effects of a changing climate are increasing the unpredictability of water, making water scarcity worse. Water retained in soil, snow, and ice—terrestrial water storage—is dwindling. Increased water scarcity disrupts societal activity.
Land System Change and Impact on Finite Resources
Land systems constitute the terrestrial component of the Earth system and consist of all processes and activities related to the human use of land, including socioeconomic, technological, and organisational investments and arrangements, as well as the benefits gained from land and the unintended social and ecological outcomes of societal activities (Verburg et al., 2013). Changes in land systems have large consequences for the local environment and human well-being and are simultaneously pervasive factors of global environmental change. Land provides vital resources to society, such as food, fuel, fibres, and many other ecosystem services that support production functions, regulate risks of natural hazards, or provide cultural and spiritual services. By using the land, society alters and modifies the quantity and quality of the provision of these services.
Land system changes are the direct result of human decision-making at multiple scales ranging from local landowners’ decisions to national-scale land use planning and global trade agreements. The aggregate impact of many local land system changes has far-reaching consequences for the Earth System feedback on ecosystem services, human well-being, and decision-making (Crossman et al., 2013). As a consequence, land system change is both a cause and consequence of socio-ecological processes.
Importance of Data
Progress in land system science depends on conceptual progress and the availability of accurate land change data. Accelerating global change and increasing global connections forces land system scientists to strive for more timely and accurate data. At the same time, our increasing knowledge of land change and the more sophisticated questions arising from that knowledge enforce the need for increased semantic depth in land use data. Data for land use change analyses are largely derived from remote sensing data, census information, or finer-scale field surveys, such as cadastres or participatory mapping. While much progress has been made in harmonising such heterogeneous data, there are multiple challenges associated with spatially, temporally, and thematically heterogeneous data sources and their inherent uncertainties (Verburg et al., 2011).
Changes in global governance increasingly affect land systems and wider revaluation of land, and in turn, this influences the wider transformations in governance and value. For example, global demand for food and biofuels has driven one of the most visible revaluations of land over the past decade, giving rise to large-scale land acquisitions by states, transnational corporations, and financial investors (Anseeuw et al., 2012).
Impact on Natural Capital
Natural capital (in essence, the major components of the land system: soil, water, vegetation, and biota) directly supplies ecosystem services. Therefore, land use and land use change decisions have a direct bearing on natural capital and ecosystem service supply (Foley et al., 2005). The impacts of these decisions typically unfold at local to regional scales, affecting local livelihoods that depend on ecosystem services and biodiversity (Wu, 2013). Trade-offs often arise between bundles of ecosystem services supplied by alternative land uses (Crossman et al., 2011). For example, land use change from natural forest to annual cropping or grazing systems may enhance the supply of food production for the ecosystem service, but this will be at the cost of several other services, such as water purification, local climate regulation, carbon sequestration, biodiversity, and habitat, and includes important cultural and spiritual value (Smith et al., 2013).
Land system science could benefit from a stronger engagement of stakeholders to develop sustainability solutions. The orientation towards sustainability has a long tradition in land system science. There is, however, a need for further engagement in using the acquired knowledge to develop and prototype sustainable land management practices and policies. Land system science is closely related to the fields of land use planning and policy. However, private landowners who manage much land do not always respond to planning and policy. Governments and organisations must develop new ways of linking science and practice to effectively create scientific solutions that contribute to long-term sustainability.
Land systems are both a cause of global environmental change and a possible powerful means of mitigation of adaptation to global environmental change.
Acceleration of biodiversity loss
Biodiversity refers to the variety of all living forms and their genetic mutations. It also includes the various interactions that these species have with the Earth’s ecosystems. Climate change and human behaviours are wiping out biodiversity at an alarming speed, and it takes several centuries for ecologies to revive from such an episode. Biodiversity is vital for many reasons to humanity. Humanity has assumed that biodiversity is eternal. Biodiverse ecosystems provide humanity with food, fresh air, clean water, medicines, fuel sources, and climate stability.
Importance of Biodiversity for Ecosystems
Plants create chemical energy stored in the form of sugar and release oxygen as a by-product through a process called photosynthesis. The energy from the sun, water from the air and soil, and carbon dioxide from the atmosphere are used in this mechanism. Sugar, known as glucose, is necessary to make starch. This is then deposited in seeds and other plant parts as a source of food available to other life forms.
Importance of Bacteria
Bacteria release enzymes are molecules that are living organisms regulating chemical reactions that break down organic matter into nutrients like phosphorus to help the plants grow. Similarly, bacteria also discharge nitrogen releasing enzymes within nodules on the plant’s roots, making nitrogen accessible to the plants for use. This happens within a nodule made out of plant cells, with the aid of the bacteria, because oxygen can damage the nitrogen-releasing enzymes. The beneficial bacteria also shield the plants from harmful phytopathogens. It does so by inducing systemic resistance through being in contact with the plant roots to trigger the plant’s defence mechanism, protecting the plant against pathogens. This is similar to the process by which a vaccine builds immunity.
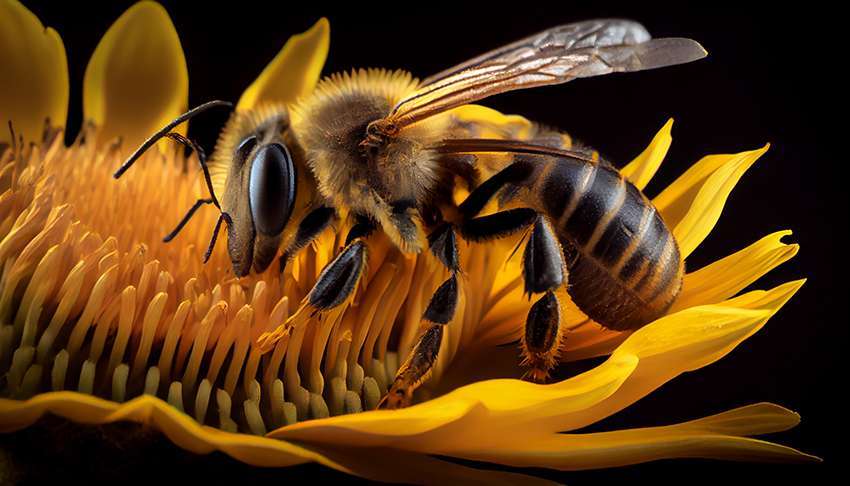
Close up of a yellow honey bee pollinating using generative AI.
Source: Freepik.
Why are pollinators important?
Pollinators are critical to sustaining the food supply for humanity through plant reproduction. Few plants can pollinate themselves. The majority of these plants rely on pollinators, along with wind and water, to reproduce. Thus, the decline in pollinators threatens the extinction of plant species as well. This endangers the food production and supply chains for humans.
Plants also absorb carbon dioxide and release oxygen. These act as natural carbon sinks, along with the soil and oceans. A carbon sink is a natural or man-made reservoir for CO2. Examples of natural carbon sinks are soil, forest, and ocean. Artificial carbon sinks could take the form of high-pressure CO2 storage deep beneath the Earth’s crust.
Major Causes of Biodiversity Loss: Slowly Losing our Non-Renewables
The Primary Reason is the Changes in Land Use:
- Urbanisation leads to habitat loss and changes in the natural environments of the species due to human encroachment.
- When forests are destroyed through human activity or natural disasters like forest fires, This releases trapped carbon dioxide into the atmosphere. This adds to the vicious cycle of climate change through greenhouse gas emissions. Extreme weather events like droughts, floods, heavy rains, tropical storms, heat waves, and fire weather are escalating in incidence and intensity. These will, in turn, make forest losses worse.
- Intense agriculture for increased food production, through irrigation and chemical fertilisers destruct the nutrients in the soil, hampering the healthy existence of the plant species. Consequently, this depletes human nutritive dietary health and well-being.
Direct Exploitation
- Direct exploitation, such as overhunting and overfishing, is harmful to biodiversity. Bycatch is where unwanted marine species get injured, die, or cannot reproduce due to their encounter with fishing equipment or vessels. This risks the breeding process to maintain the stock of overfished species. Also, damaging the corals and sponges can disturb the fish habitat. Removing some species that are prey to others can be detrimental.
- Wildlife trafficking, for economic benefits, can threaten the extinction of endangered animals and plants. These species, alive or dead, are used for food, rugs, handbags, shoes, medicines, status symbols, décor, furniture, and ornamental purposes. The collapse in the ecosystems can be deleterious to humankind through cascading effects such as extreme weather and the spreading of infectious diseases.
Other Factors
- Climate change is another major contributor to biodiversity loss. It induces extreme weather patterns and changes in rainfalls that lead to alterations in the ecosystem. Droughts, floods, and heatwaves all affect the various plants and other living forms.
- Air, water, and soil pollution caused by fertilisers, pesticides, oil spillage, greenhouse gas emissions, and plastic waste seriously threaten all species that depend on fresh air, clean water, and nutritive soil for crop and food production.
- Invasive alien species and infectious diseases can spread through trade and travel, threatening the survival of species, including humans, and aggravate biodiversity loss.
Chemical Pollution and Impact on Finite Resources
Chemical pollution is the contamination of our environment with substances that are not normally there. This impacts the delicate equilibrium of Earth’s ecosystem. Pollution can affect our planet through the air we breathe, the soil we farm, and the water we use. Chemical pollutants can also transfer from one medium to another. For instance, air pollution carries toxins which infect the soil or water it comes into contact with.
Air Pollutants
There are six types of air pollutants. They include particulate pollution (also known as particulate matter), sulphur oxides, lead, nitrogen oxides, and ground-level ozone. Sulphur dioxide can irritate the respiratory tracts, which raises the possibility of infections. Lead exposure can result in high blood pressure and problems with the brain, kidneys, and reproductive system. Low concentrations of nitrogen oxides in the air can irritate your eyes, nose, throat, and lungs, making you cough and possibly feel exhausted, nauseous, and fatigued.
Soil Pollutants
Soil pollution is when high amounts of harmful compounds are in the soil. Significant soil pollution has been brought on by mining, agriculture, and waste disposal. Heavy metals like cadmium, mercury, and lead can degrade soil fertility by reducing the diversity of microorganisms that maintain it. The condition of the soil can influence both biodiversity and food production for the rising population.
Chemical pollution is dangerous to human health. Cadmium may accumulate in the kidneys. Kidney disease and frail bones can develop due to exposure from low quantities of cadmium in food, water, and the environment. It is believed that cadmium causes cancer. Even small amounts of mercury can adversely affect the neurological, digestive, and immunological systems, as well as the lungs, kidneys, skin, and eyes.
Water Pollutants
When chemicals or other things enter the aquatic ecosystem or when natural chemicals accumulate in excess in bodies of water, water pollution occurs. Chemical pollutants can linger in the environment for a very long time and include arsenic, mercury, selenium, and lead. They might hinder the development of aquatic life, harming it. This primarily enters the maritime environment through oil spills and may negatively impact birds, flora, and marine life. Arsenic also causes cancer, which affects mostly the skin and the lungs, but can cause other forms of cancer. Severe issues, such as breathing difficulties, tremors, kidney failure, heart attacks, and heart failure, can result from extremely high selenium doses.
moving forward
Simple solutions in everyday life are incredibly important in conserving these finite resources. For instance, limiting water use, preventing water leakage, using grey water to water plants, and so on, are some of the basic practical ways to save fresh water, one of the most essential non-renewables. Reducing shower time by one or two minutes can save about 500 litres of water each month.
Developing innovative substitutes and reducing the use of rare earth minerals are also critical to preserving non-renewables. For example, scientists are researching a rare-earth-free mineral known as tetrataenite. This has advanced permanent magnet properties that can substitute magnets made from rare earth materials.
Reusing and recycling materials such as textiles, plastics, glass, metals, and e-waste can also minimise resource depletion. A regenerative economy that incentivises the reuse of goods instead of discarding them and then extracting new resources is crucial. The economy can then absorb all kinds of waste again into its system to repurpose or recycle. This will not only protect non-renewables efficiently but also create innovative industries and new employment with fresh skill sets.
Transitioning from finite resources, such as fossil fuel-based energy sources, to renewables, like wind and solar, is another vital solution. Integrating different systems, like water desalination along with renewable energy and storage energy, is encouraging. It can relieve water stress while reducing greenhouse emissions.
A Thrivable Framework
To learn more about how THRIVE Project is researching, educating and advocating for a future beyond sustainability, visit our website. You can follow our informative blog and podcast series and learn about our regular live webinars featuring expert guests in the field. Sign up for our newsletter for regular updates.