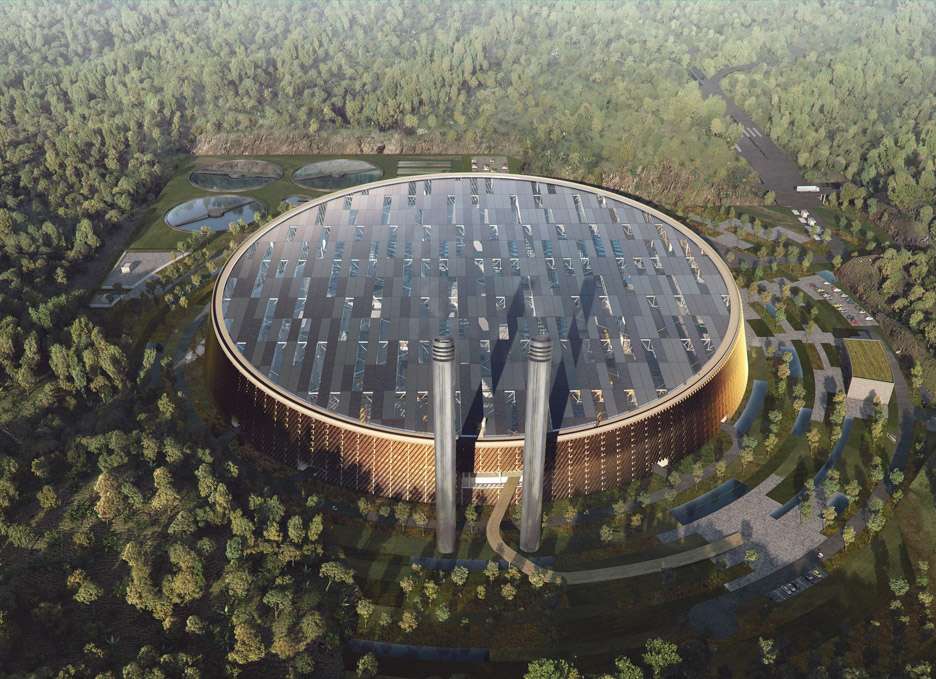
Source: De Zeen
Decades of linear consumption habituate consumerism, valuing raw material products, causing waste in landfills and oceans (Anghel & Hristea, 2018). Unfortunately, in this prevailing fashion of overconsumption, the emphasis is on a push for purchasing products, often disregarding their long-term sustainability. Consequently, the discarded waste ends up polluting our precious land and marine ecosystems, posing significant challenges for the planet (Anghel & Hristea, 2018). Accordingly, waste-to-energy technologies convert discarded waste into energy sources instead of allowing it to harm the environment. Advanced waste-to-energy technologies don’t stop at converting waste into energy- they promote eco-friendly consumption and support a circular economy (Boloy, 2021).
What are waste-to-energy technologies?
Basically, waste-to-energy technologies minimise environmental impact while generating renewable energy, promoting sustainable waste management (Tabasová, 2012). There are many examples and essential factors that enhance the sustainability of waste-to-energy solutions.
Anaerobic Digestion & Reduction of Landfill Waste
Anaerobic digestion (AD) breaks down organic waste without oxygen, producing biogas and nutrient-rich digestate (EPA, 2023). It diverts organic waste from landfills, preventing methane production (a potent greenhouse gas)(Aworanti, 2023). At the AD facility, biogas is generated for heating, electricity, or vehicle fuel. Evidently, these technologies reduce waste sent to landfills, minimising environmental impacts linked to landfill disposal (Aworanti, 2023).
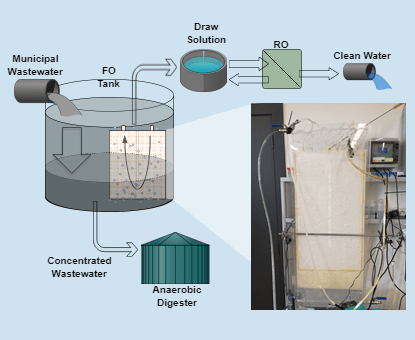
Source: MDPI
Thermochemical Conversion & Renewable Energy Generation
Thermochemical conversion uses heat and chemical reactions to transform biomass and waste into biofuels, syngas, and chemicals (Lee, 2022). High-temperature reactions (incineration, gasification, and pyrolysis) transform feedstock into valuable energy and chemical compounds. Accordingly, intermittent renewable energy disrupts single-source supply, aiding development in developing countries. This reduces reliance on fossil fuels and contributes to the transition to a low-carbon energy system.
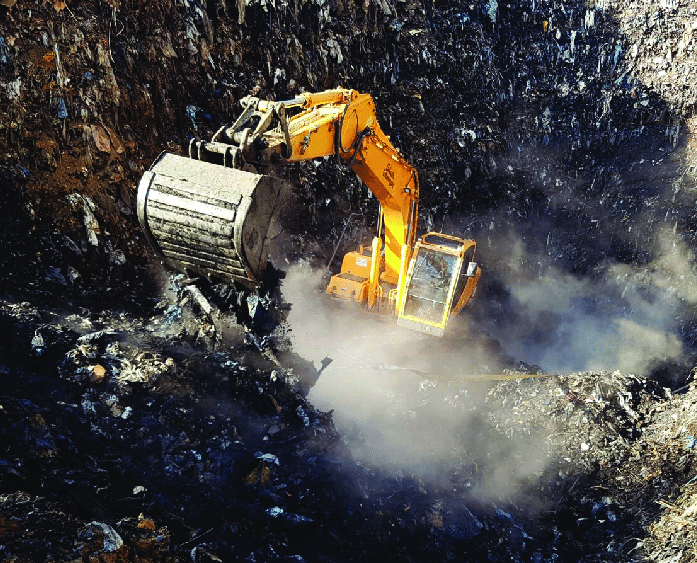
Source: Eurowaste SRL
Landfill Mining & Resource Recovery
Landfill mining (LFM) excavates and reprocesses old landfill sites to recover valuable resources, reducing the need for virgin materials (Goli, 2022). Additionally, LFM recovers energy from decomposing waste using technologies like landfill gas capture and energy recovery systems (Pitak, 2023).
Sustainable Waste-to-Energy Technologies and Water Management
THRIVE believes that sustainable technology is something that takes all possible factors into consideration and serves a variety of purposes simultaneously. Significantly, one such example is bioenergy, which is derived from organic sources such as biomass or biogas and can be utilised for wastewater treatment processes. Moreover, this also falls under ‘modern‘ technology. Anaerobic digestion of wastewater sludge can produce biogas, which can be used for heat and power generation. In turn, this reduces the carbon footprint of wastewater treatment plants and provides cost-effective solutions for wastewater management, supporting a circular economy (Pasciucco, 2023).
The traditional renewable sources that we know of today can contribute to water management too. Emphatically, solar energy can be utilised for power water treatment systems, particularly in areas with limited access to electricity (Horváth, 2022). Solar-powered water treatment technologies, such as solar stills/hydro panels and pumps can provide clean drinking water by harnessing the sun’s energy (SOURCE Water, 2023). Similarly, these systems reduce reliance on fossil fuels, lower operational costs, and provide sustainable solutions for water treatment in off-grid or remote locations (Horváth, 2022).
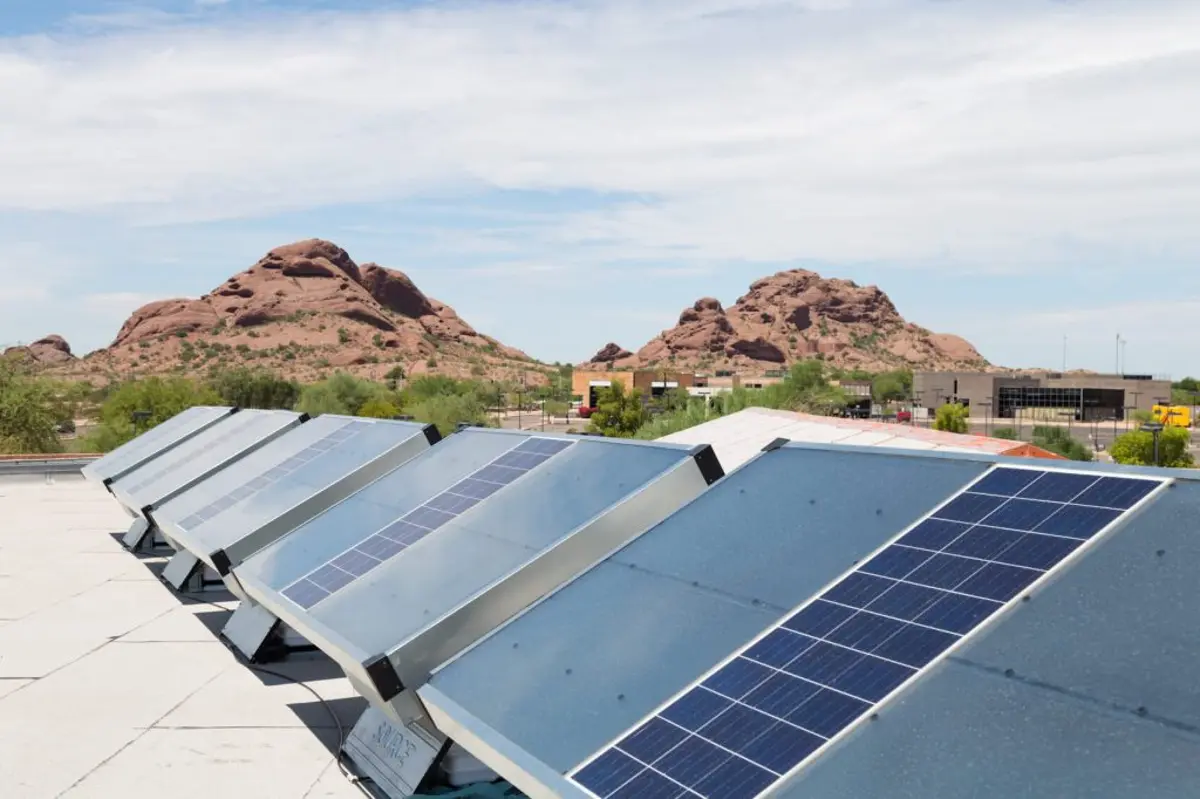
Source: Clean Technica
Significantly, hydropower provides more than 15% of the world’s electricity. Integrated water management strategies can optimise the operation of hydropower systems to balance energy production and water supply needs. Moreover, this involves managing reservoir levels, releasing water for irrigation or downstream ecosystems, and coordinating hydropower generation with peak energy demand. Interestingly, such approaches contribute to sustainable water management by ensuring the efficient use of water resources and minimising impacts on aquatic systems.
“The health and well-being of our lands, waters and wildlife is always the priority.”
Chief Bill Cranmer
countries that have endeavoured to utilise waste-to-energy technologies
Sweden
Sweden is one of the leading countries in waste-to-energy implementation. Apparently, it has developed a highly efficient waste management system that prioritises waste prevention, recycling, and waste-to-energy conversion. Significantly, more than 50% of municipal solid waste in Sweden is used for energy generation, deeming it renewable (Igini, 2022).
Singapore
Singapore has adopted innovative waste management practices and has integrated waste-to-energy as part of its sustainable waste management strategy (CNBC International, 2021). Additionally, overcoming the challenge of limited land space, the country implemented advanced waste-to-energy facilities that incinerate waste, generate electricity and recover heat for various purposes. Singapore’s waste-to-energy plants help reduce reliance on landfilling and contribute to the country’s renewable energy goals (San, 2023
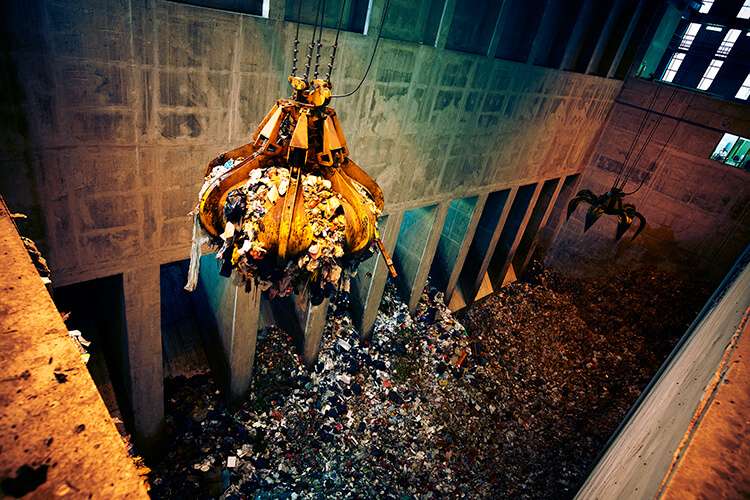
Source: Keppel Infrastructure Trust
Interestingly, ‘scrap selling’ is an age-old trade in India. Startups are now making it more convenient by developing mobile apps for the systematic collection of household scraps. Significantly, India has also been exploring the production of refuse-derived fuel (RDF) from non-recyclable combustible waste. RDF is produced by processing and shredding solid waste to remove recyclable materials, resulting in a high-calorific fuel. Moreover, this fuel can be used in industrial boilers and cement kilns to replace fossil fuels, thereby reducing greenhouse gas emissions and promoting resource recovery (Wastech, 2022).
Currently, Egypt uses 4.2 million tonnes of municipal solid waste for waste-to-energy technologies. Interestingly, Egypt has been exploring public-private partnerships to attract investment and expertise in waste-to-energy projects, such as the Benban Solar Park near southern city of Aswan. By partnering with private entities, Egypt aims to accelerate the development and implementation of innovative waste-to-energy technologies.
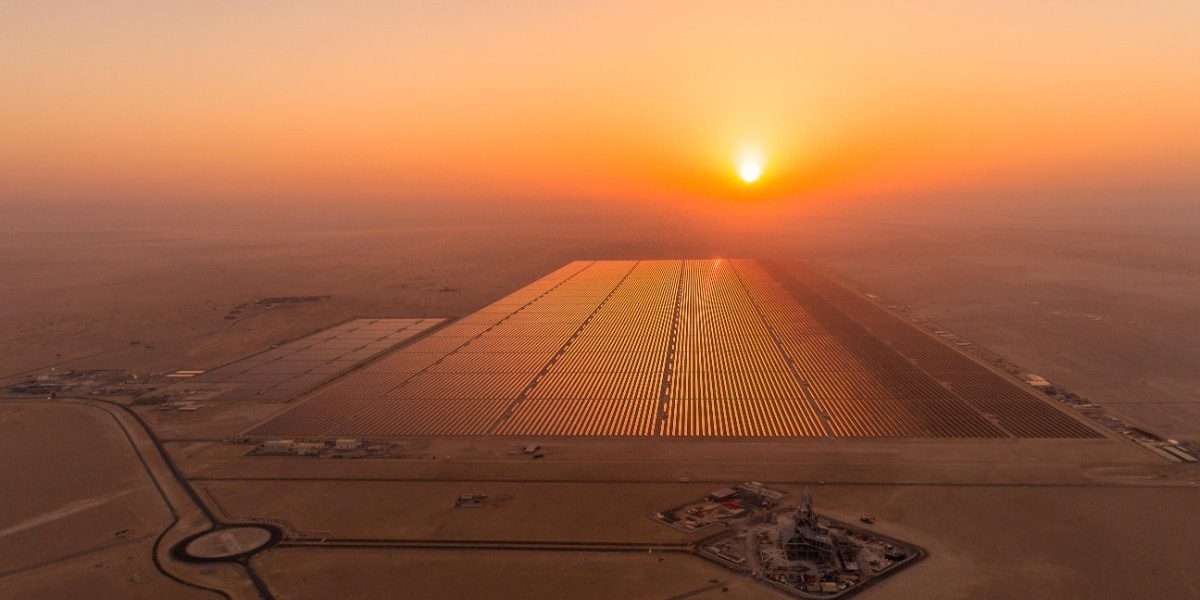
Source: African Vibes
Why Are Waste-to-Energy Technologies Important for Sustainable Energy and Water Management?
In a world facing the challenges of waste management, energy security, and water scarcity, waste-to-energy technologies offer innovative solutions that can help address these pressing issues. Emphatically, one of the primary reasons waste-to-energy technologies are important for sustainable energy management is their contribution to renewable energy generation. By converting waste materials into usable forms of energy such as electricity and heat, there’s a reduction in reliance on fossil fuels. The revision of a target for source recycling to 58% will lead to more significant reductions in greenhouse gas emissions. This not only helps mitigate greenhouse gas emissions but also contributes to energy diversification and security (ESWET, 2022).
Furthermore, waste-to-energy technologies offer the potential for resource recovery and circular economy principles. These technologies can contribute to water management by enabling resource recovery (ESWET, 2022). Certain processes, such as anaerobic digestion allow for the extraction of water from waste streams. Furthermore, this recovered water can be treated and reused, reducing freshwater demand and promoting water conservation.
Moving Forward
In summary, both individuals and governments can take steps to contribute to global waste-to-energy initiatives:
Reduce, Reuse, and Recycle
Minimise waste generation by opting for reusable items. Recycling materials whenever possible, and supporting recycling initiatives in the community can make a massive difference.
Promote Responsible Consumption:
Make informed choices by favouring products with minimal packaging. Also, opt for durable and reusable items, and support brands that prioritise sustainability.
Develop Waste Management Policies:
Governments formulate comprehensive waste management policies that promote waste-to-energy technologies. Setting recycling targets, and incentivising sustainable waste management practices will prove helpful.
Implement Extended Producer Responsibility (EPR):
Enforcing extended producer responsibility (EPR) programs. This will ensure that manufacturers take responsibility for the entire lifecycle of their products. Additionally, it promotes the adoption of sustainable packaging and disposal practices.
achieving the United Nations Sustainability Development Goals (SDGs)
In short, waste-to-energy technologies contribute to SDG 7 by providing affordable and clean energy. Converts waste materials into usable forms of energy such as electricity, heat, or biofuels, promoting renewable energy generation. Waste-to-energy technologies also help achieve SDG 11 Sustainable Cities and Communities by promoting sustainable waste management in cities. It minimises waste volume and environmental impacts of landfills and promotes sustainable resource use (Sanfo, 2022).
Waste-to-energy aligns with SDG12: Responsible Consumption and Production. Moreover, this SDG encourages waste reduction, recycling, and resource recovery, contributing to a circular economy approach. Lastly, Waste-to-energy helps address SDG 13 Climate Action by mitigating greenhouse gas emissions.
A Thrivable Framework
The Thrive Project invests interest in issues fundamental to the longevity of our society on this planet. THRIVE aims to research, advocate and bring awareness among larger audiences and governments on topics relating to waste and methods to reduce and possibly eliminate the burden on landfills, investigating proper water management.
Ultimately, sustainability ensures survival, but thrivability goes beyond mere sustainability. It encapsulates the vision to live and build a world where every individual not only survives but genuinely thrives. Conversely, waste-to-energy methods assist in implementing a circular economy. By adapting and promoting responsible consumption, we as a society, can help make the transition possible. THRIVE promotes sustainable solutions for preventing disaster and enabling flourishing societies.
THRIVE framework recognises that we, unfortunately, live in a finite world with unrealistic demands that threaten our long-term livelihood on this planet. THRIVE framework focuses on shifting from a linear consumption model to a circular one. THRIVE framework looks for solutions to maintain the scale of finite resources in the desired range and ultimately bring down the quantity of waste. Safeguarding human well-being in all domains is paramount to THRIVE’s mission.
To learn more about how The THRIVE Project is researching, educating and advocating for a future beyond sustainability, visit our website. You can follow our informative blog and podcast series. Learn about our regular live webinars featuring expert guests. Sign up for our newsletter for regular updates.